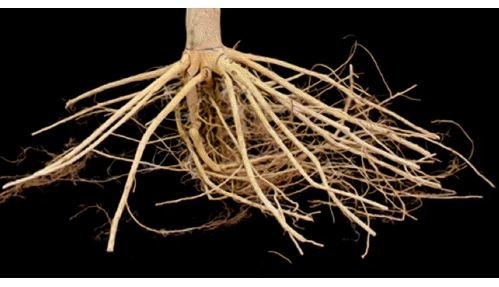
Root systems are fundamental to plant survival, serving as the primary interface between plants and their soil environment. They anchor plants, absorb water and nutrients, store energy, and facilitate interactions with soil microorganisms. Root systems vary widely in structure, depth, spread, and function, shaped by evolutionary adaptations to diverse habitats, climates, and ecological roles.
Primary Types of Root Systems
Root systems are broadly classified based on their origin, structure, and growth patterns. The three primary types—taproot, fibrous, and adventitious—form the foundation for understanding plant root diversity. Each type is adapted to specific environmental conditions and serves distinct functional roles.
Taproot System
The taproot system features a single, prominent central root (the taproot) that grows vertically downward, with smaller lateral roots (secondary and tertiary roots) branching off horizontally. Originating from the radicle during seed germination, the taproot is typically thick and elongated, penetrating deep into the soil.
Morphology: The taproot is robust, often conical or cylindrical, with a diameter larger than lateral roots. Lateral roots are thinner, spreading outward to form a network, though less extensive than in fibrous systems. The taproot may develop a woody texture in perennials.
Functions:
- Anchorage: Provides strong anchoring, resisting wind and physical stress, ideal for tall plants like trees.
- Water and Nutrient Absorption: Accesses deep water and mineral reserves, critical in dry or nutrient-poor soils.
- Storage: Stores carbohydrates, water, and nutrients, supporting regrowth in perennials or during drought.
Adaptations: - Deep penetration (up to 5–10 m in some species) suits arid environments or rocky soils.
- Storage capacity supports survival in seasonal climates (e.g., biennials like carrots).
- Secondary thickening in woody plants enhances structural support.
Examples: - Carrot (Daucus carota): Fleshy taproot stores sugars, used as a food crop.
- Dandelion (Taraxacum officinale): Long taproot aids survival in compacted soils.
- Oak (Quercus spp.): Deep taproot supports massive canopy in mature trees.
Ecological Significance: Taproot systems stabilize soil on slopes, reducing erosion, and access deep groundwater, benefiting plants in drought-prone regions. In agriculture, taproot crops (e.g., beets, radishes) are harvested for their storage roots, while their deep roots improve soil structure.
Limitations: Slow establishment in shallow or waterlogged soils; vulnerable to damage during transplanting.
Fibrous Root System
The fibrous root system consists of numerous, thin, thread-like roots of similar size, forming a dense, mat-like network near the soil surface. Originating from the stem base or radicle, it lacks a dominant central root.
Morphology: Roots are fine (0.1–2 mm diameter), highly branched, and spread horizontally, often within the top 30 cm of soil. They form a diffuse, fibrous mass, maximizing surface area for absorption.
Functions:
- Absorption: Extensive surface area enhances water and nutrient uptake, ideal for fertile, moist soils.
- Anchorage: Dense network stabilizes plants, especially in loose or sandy soils.
- Soil Binding: Prevents erosion by holding soil particles together.
Adaptations: - Shallow, wide spread (up to 1–2 m radius) suits wet or nutrient-rich environments.
- Rapid growth enables quick establishment in annuals or grasses.
- Mycorrhizal associations enhance nutrient uptake (e.g., phosphorus) in poor soils.
Examples: - Wheat (Triticum aestivum): Fibrous roots support rapid nutrient absorption in croplands.
- Grass (Poa pratensis): Dense roots stabilize lawns and pastures.
- Onion (Allium cepa): Fibrous roots form a shallow, spreading network.
Ecological Significance: Fibrous roots are critical in grasslands and prairies, preventing soil erosion and supporting soil microbial communities. In agriculture, they promote soil health by adding organic matter upon decomposition. Their shallow nature makes them efficient in rain-fed systems but vulnerable to drought.
Limitations: Limited access to deep water; susceptible to drying in arid conditions or competition in dense plantings.
Adventitious Root System
Adventitious roots arise from non-root tissues, such as stems, leaves, or nodes, rather than the radicle. Common in monocots and some dicots, they form diverse structures depending on environmental cues.
Morphology: Roots vary from thin and fibrous to thick and fleshy, often growing in clusters from stems or aerial tissues. Their arrangement depends on the plant’s habitat (e.g., aerial, soil-bound, or aquatic).
Functions:
- Anchorage: Supports climbing or sprawling plants, stabilizing them against gravity or wind.
- Absorption: Absorbs water and nutrients, especially in epiphytes or flood-prone plants.
- Propagation: Facilitates vegetative reproduction in cuttings or layering.
Adaptations: - Versatile origins allow growth in response to flooding, injury, or aerial exposure.
- Specialized forms (e.g., prop roots, aerial roots) suit unique habitats like mangroves or rainforests.
- Rapid development aids survival in unstable environments.
Examples: - Corn (Zea mays): Prop roots from stem nodes provide additional support.
- Ivy (Hedera helix): Aerial roots cling to surfaces for climbing.
- Orchid (Orchidaceae): Aerial roots absorb moisture from air in epiphytic species.
Ecological Significance: Adventitious roots enable plants to colonize challenging environments, such as wetlands, cliffs, or tree canopies, enhancing biodiversity. In agriculture, they support clonal propagation (e.g., sugarcane) and stabilize soils in flood-prone areas.
Limitations: May require high humidity or specific conditions for optimal function; less effective in deep, dry soils.
Specialized and Modified Root Systems
Beyond the primary types, plants develop specialized or modified root systems as adaptations to extreme environments, unique ecological niches, or specific physiological needs. These roots often derive from taproot, fibrous, or adventitious systems but exhibit distinct structural or functional modifications.
Storage Roots
Storage roots are swollen, fleshy roots that store carbohydrates, water, or nutrients, derived from taproots or adventitious roots. They support regrowth, drought survival, or reproduction in perennials and biennials.
Morphology: Enlarged, tuberous, or conical, with starchy or watery tissues (e.g., parenchyma). Examples include thickened taproots (carrots) or adventitious tuberous roots (sweet potatoes).
Functions:
- Storage: Accumulates energy reserves for flowering, fruiting, or regrowth.
- Survival: Sustains plants during dormancy or environmental stress.
- Propagation: Some (e.g., dahlia tubers) produce new shoots for cloning.
Adaptations: - High starch or water content suits arid or seasonal climates.
- Thick, protective skin reduces desiccation and pathogen entry.
- Regenerative capacity supports vegetative propagation.
Examples: - Sweet Potato (Ipomoea batatas): Tuberous adventitious roots store starches.
- Beet (Beta vulgaris): Fleshy taproot stores sugars.
- Cassava (Manihot esculenta): Tuberous roots store carbohydrates for food security.
Ecological Significance: Storage roots are vital in agriculture, providing staple crops with high nutritional value. Ecologically, they support plant survival in harsh climates, contributing to ecosystem resilience. In restoration, they stabilize soils and provide food for herbivores.
Applications: Major food crops; used in bioremediation for their soil-binding capacity.
Prop Roots
Prop roots are adventitious roots growing from stems or branches, extending downward to provide additional anchorage. Common in tall or flood-prone plants, they resemble stilts or braces.
Morphology: Thick, woody, or fibrous, often forming a tripod-like structure from the lower stem or aerial branches. They may penetrate soil or remain aerial.
Functions:
- Anchorage: Supports tall or top-heavy plants against wind or flooding.
- Absorption: Some absorb water and nutrients in wet environments.
- Stability: Distributes weight in shallow or unstable soils.
Adaptations: - Buttress-like growth enhances stability in wetlands or coastal zones.
- Lignified tissues resist decay in waterlogged conditions.
- Secondary roots from props increase nutrient uptake.
Examples: - Maize (Zea mays): Prop roots from stem nodes stabilize tall stalks.
- Mangrove (Rhizophora spp.): Stilt-like prop roots anchor in tidal mudflats.
- Banyan (Ficus benghalensis): Aerial prop roots form new trunks.
Ecological Significance: Prop roots are critical in coastal ecosystems, preventing erosion and creating habitats for marine life in mangroves. They enable plants to thrive in dynamic environments, enhancing biodiversity. In agriculture, they support high-yielding crops like corn.
Applications: Used in coastal restoration and erosion control; studied for biomechanical engineering.
Aerial Roots
Aerial roots are adventitious roots growing above ground, often in epiphytes or climbers, to absorb moisture, anchor plants, or photosynthesize. They vary from thin and wiry to thick and woody.
Morphology: Velamen (spongy tissue) in some (e.g., orchids) absorbs atmospheric water; others are adhesive or climbing (e.g., ivy). May be green for photosynthesis in some species.
Functions:
- Absorption: Captures moisture and nutrients from air or rain.
- Anchorage: Secures epiphytes to host trees or climbers to surfaces.
- Photosynthesis: Green aerial roots produce energy in low-soil environments.
Adaptations: - Velamen tissue maximizes water retention in humid, rain-scarce conditions.
- Adhesive tips or root hairs grip bark or rock surfaces.
- Chlorophyll in roots supports energy needs in canopy-dwelling plants.
Examples: - Orchid (Cattleya spp.): Velamen-covered roots absorb rainforest humidity.
- Poison Ivy (Toxicodendron radicans): Aerial roots cling to trees or walls.
- Monstera (Monstera deliciosa): Aerial roots support climbing vines.
Ecological Significance: Aerial roots enable epiphytes to colonize forest canopies, increasing tropical biodiversity. They contribute to nutrient cycling by trapping organic debris. In horticulture, they enhance ornamental appeal and support propagation.
Applications: Used in air layering; critical in rainforest conservation and urban greening.
Buttress Roots
Buttress roots are large, flattened, plank-like roots extending from the base of tree trunks, providing structural support. Derived from taproots or adventitious roots, they are common in tropical trees.
Morphology: Broad, triangular, extending several meters from the trunk, often above ground. Woody and lignified, they form a star-like pattern around the tree base.
Functions:
- Anchorage: Stabilizes tall trees in shallow, nutrient-poor tropical soils.
- Support: Counteracts wind or gravity stress in high-rainfall areas.
- Nutrient Uptake: Secondary roots from buttresses absorb surface nutrients.
Adaptations: - Wide spread (up to 10 m) compensates for shallow soil depth.
- Lignified tissues resist fungal decay in humid tropics.
- Surface positioning maximizes nutrient capture in rain-leached soils.
Examples: - Kapok (Ceiba pentandra): Massive buttresses support towering rainforest trees.
- Mahogany (Swietenia macrophylla): Buttresses stabilize in tropical forests.
- Fig (Ficus spp.): Buttresses enhance longevity in wet soils.
Ecological Significance: Buttress roots create microhabitats for fungi, insects, and epiphytes, boosting rainforest biodiversity. They prevent soil erosion on slopes and support massive carbon sinks. In forestry, they complicate logging but enhance tree stability.
Applications: Studied for biomechanical design; used in reforestation to stabilize tropical soils.
Pneumatophores
Pneumatophores are upward-growing roots, often adventitious, that facilitate gas exchange in waterlogged or anaerobic soils. Common in mangrove and swamp plants, they emerge from submerged roots.
Morphology: Vertical, peg-like or conical, with lenticels (pores) for oxygen intake. May reach 20–30 cm above soil or water, often in dense clusters.
Functions:
- Gas Exchange: Supplies oxygen to submerged roots in low-oxygen soils.
- Anchorage: Stabilizes plants in muddy or tidal environments.
- Nutrient Absorption: Some pneumatophores take up nutrients from sediment.
Adaptations: - Lenticels and aerenchyma (air-filled tissues) enhance oxygen transport.
- Dense clustering resists tidal currents and wave action.
- Salt-filtering mechanisms in mangroves cope with saline conditions.
Examples: - Black Mangrove (Avicennia germinans): Pneumatophores dot tidal flats.
- Bald Cypress (Taxodium distichum): Knee-like pneumatophores in swamps.
- White Mangrove (Laguncularia racemosa): Pneumatophores aid gas exchange.
Ecological Significance: Pneumatophores enable plants to thrive in wetlands, supporting critical ecosystems like mangroves that protect coastlines, sequester carbon, and provide fish nurseries. They enhance soil aeration, benefiting microbial communities.
Applications: Essential in wetland restoration; studied for flood-tolerant crop development.
Contractile Roots
Contractile roots are specialized roots that physically pull plant parts (e.g., bulbs, corms) deeper into the soil through contraction. Common in geophytes and some perennials, they derive from taproots or adventitious roots.
Morphology: Thick, fleshy, with longitudinal wrinkles from contraction. They shrink vertically, anchoring bulbs or stems at optimal soil depths.
Functions:
- Positioning: Adjusts plant depth for protection from heat, cold, or grazing.
- Anchorage: Secures bulbs or corms in loose soils.
- Storage: Often stores nutrients for regrowth.
Adaptations: - Contractile tissues with specialized cells shorten roots over time.
- Rapid response to seasonal cues ensures proper positioning.
- Thick structure resists soil resistance during pulling.
Examples: - Tulip (Tulipa spp.): Contractile roots pull bulbs deeper annually.
- Crocus (Crocus spp.): Roots adjust corm depth for winter protection.
- Oxalis (Oxalis spp.): Contractile roots reposition bulbils.
Ecological Significance: Contractile roots protect geophytes in harsh climates, ensuring survival in grasslands or Mediterranean ecosystems. They support horticultural propagation by maintaining bulb health. In ecology, they stabilize soils in disturbed areas.
Applications: Used in ornamental gardening; studied for plant biomechanics.
Haustorial Roots (Parasitic Roots)
Haustorial roots are specialized roots of parasitic plants that penetrate host plants to extract water, nutrients, or photosynthates. Derived from taproots or adventitious roots, they form connections called haustoria.
Morphology: Thin, penetrating structures with haustoria that invade host vascular tissues. May be primary (direct to host) or secondary (branching within host).
Functions:
- Nutrient Extraction: Absorbs resources from host xylem or phloem.
- Attachment: Secures parasite to host, ensuring sustained contact.
- Survival: Supports parasitic plants lacking photosynthesis (e.g., dodder).
Adaptations: - Chemical cues detect host plants for targeted penetration.
- Haustoria form tight seals to minimize host damage while maximizing uptake.
- Rapid growth ensures quick host attachment in hemiparasites or holoparasites.
Examples: - Dodder (Cuscuta spp.): Thread-like roots parasitize host stems.
- Mistletoe (Viscum album): Haustorial roots invade tree branches.
- Broomrape (Orobanche spp.): Roots parasitize crop roots underground.
Ecological Significance: Haustorial roots regulate plant communities by controlling dominant species, influencing biodiversity. In agriculture, parasitic plants reduce crop yields (e.g., Striga on maize), necessitating resistant varieties or control measures. They contribute to nutrient cycling in natural ecosystems.
Applications: Studied for biocontrol of invasive plants; challenges in crop protection.
Mycorrhizal Roots
Mycorrhizal roots are symbiotic associations between plant roots (taproot, fibrous, or adventitious) and fungi, enhancing nutrient and water uptake. Common in 80–90% of terrestrial plants, they involve arbuscular, ectomycorrhizal, or ericoid fungi.
Morphology: Roots appear normal but host fungal hyphae or sheaths; arbuscules or mantles form within or around root cells. Fibrous roots often dominate due to high surface area.
Functions:
- Nutrient Uptake: Fungi absorb phosphorus, nitrogen, or micronutrients for plants.
- Water Absorption: Hyphae extend root reach in dry or poor soils.
- Protection: Fungi deter pathogens and improve soil structure.
Adaptations: - Fungal hyphae increase effective root surface area by 10–100 times.
- Chemical signaling ensures mutualistic exchange (e.g., sugars for nutrients).
- Specific fungal partners suit plant needs (e.g., ectomycorrhizae for trees).
Examples: - Pine (Pinus spp.): Ectomycorrhizal roots enhance forest growth.
- Soybean (Glycine max): Arbuscular mycorrhizae boost phosphorus uptake.
- Heath (Erica spp.): Ericoid mycorrhizae thrive in acidic soils.
Ecological Significance: Mycorrhizal roots drive nutrient cycling, supporting forest, grassland, and agricultural ecosystems. They enhance plant resilience to drought, salinity, and disease, reducing fertilizer needs. In restoration, they improve soil health and plant establishment.
Applications: Used in sustainable agriculture; critical in reforestation and soil remediation.
Nodulated Roots
Nodulated roots are specialized roots (often fibrous or adventitious) hosting nitrogen-fixing bacteria (e.g., Rhizobium, Frankia) in nodules, enabling nitrogen conversion for plant use. Common in legumes and some non-legumes.
Morphology: Roots bear spherical or elongated nodules (1–10 mm), formed by cortical cells housing bacteria. Nodules are pinkish due to leghemoglobin, which regulates oxygen.
Functions:
- Nitrogen Fixation: Converts atmospheric nitrogen to ammonia for plant growth.
- Nutrient Supply: Enhances soil fertility by releasing nitrogen compounds.
- Symbiosis: Bacteria receive sugars from plants in exchange for nitrogen.
Adaptations: - Chemical signals (flavonoids) attract specific bacteria for nodule formation.
- Nodules protect bacteria in low-oxygen environments for efficient fixation.
- Rapid nodule development responds to nitrogen-deficient soils.
Examples: - Pea (Pisum sativum): Nodulated roots enrich agricultural soils.
- Alder (Alnus spp.): Frankia nodules fix nitrogen in temperate forests.
- Soybean (Glycine max): Rhizobium nodules support high-protein crops.
Ecological Significance: Nodulated roots improve soil fertility, reducing reliance on synthetic fertilizers in agriculture. They support succession in nutrient-poor soils, aiding ecosystem recovery. In agroforestry, they enhance companion planting.
Applications: Used in crop rotation, green manures, and ecological restoration.
Climbing Roots
Climbing roots are adventitious roots that enable plants to ascend surfaces like trees, rocks, or walls, often in vines or epiphytes. They provide anchorage and sometimes absorb moisture.
Morphology: Short, adhesive, or hook-like, with root hairs or sticky tips for grip. Often fibrous, they grow from stem nodes or internodes.
Functions:
- Anchorage: Secures plants to vertical or unstable surfaces.
- Absorption: Some take up water or nutrients from bark or debris.
- Climbing: Facilitates access to sunlight in shaded environments.
Adaptations: - Adhesive secretions or root hairs maximize surface adhesion.
- Rapid growth responds to contact stimuli (thigmotropism).
- Flexible tissues resist mechanical stress from wind or host movement.
Examples: - English Ivy (Hedera helix): Climbing roots grip walls and trees.
- Vanilla (Vanilla planifolia): Roots anchor to host trees in rainforests.
- Philodendron (Philodendron spp.): Roots support climbing houseplants.
Ecological Significance: Climbing roots increase vertical niche diversity in forests, supporting epiphytic communities. They contribute to canopy ecosystems by trapping debris. In urban settings, they enhance aesthetics but may damage structures.
Applications: Used in vertical gardening; studied for bio-inspired adhesives.
Brace Roots
Brace roots are adventitious roots similar to prop roots, growing from lower stem nodes to provide additional support, often in tall monocots or flood-prone plants. They differ by their less pronounced, fibrous nature.
Morphology: Thin, fibrous, or slightly woody, forming a shallow, radiating network from the stem base. They penetrate soil or remain partially aerial.
Functions:
- Anchorage: Stabilizes plants in windy or wet conditions.
- Absorption: Supplements nutrient and water uptake in shallow soils.
- Support: Prevents lodging in tall crops or grasses.
Adaptations: - Rapid growth responds to mechanical stress or flooding.
- Fibrous structure maximizes soil contact in loose substrates.
- Secondary thickening in some species enhances durability.
Examples: - Sugarcane (Saccharum officinarum): Brace roots prevent toppling.
- Sorghum (Sorghum bicolor): Roots support drought-tolerant crops.
- Pandanus (Pandanus tectorius): Roots stabilize coastal plants.
Ecological Significance: Brace roots enhance crop resilience in agriculture, reducing yield losses from lodging. They stabilize soils in wetlands or coastal zones, supporting biodiversity. In ecology, they aid succession in disturbed habitats.
Applications: Critical in high-yield agriculture; used in erosion control.
Additional Specialized Root Systems
Some root systems are highly specialized, occurring in specific plant groups or extreme environments. These are less common but critical for understanding plant adaptability.
Coralloid Roots
Coralloid roots are short, coral-like, adventitious roots hosting nitrogen-fixing cyanobacteria (e.g., Nostoc) in cycads and some gymnosperms. They grow near the soil surface or slightly above ground.
Morphology: Branched, nodular, with a rough, coral-like texture, containing cyanobacterial zones for nitrogen fixation. Often yellowish or green due to symbiotic algae.
Functions:
- Nitrogen Fixation: Supplies nitrogen in nutrient-poor soils.
- Absorption: Limited water and nutrient uptake due to specialized role.
- Symbiosis: Supports cyanobacteria with sugars and protection.
Adaptations: - Precoralloid structures attract cyanobacteria before nodule formation.
- Surface positioning maximizes light for photosynthetic cyanobacteria.
- Protective tissues resist desiccation in arid conditions.
Examples: - Cycad (Cycas revoluta): Coralloid roots enhance survival in sandy soils.
- Zamia (Zamia integrifolia): Roots support nitrogen fixation in tropics.
- Bowenia (Bowenia spectabilis): Roots aid nutrient cycling in forests.
Ecological Significance: Coralloid roots enable cycads to thrive in poor soils, contributing to nutrient cycling in tropical and subtropical ecosystems. They support relict plant lineages, preserving biodiversity. In horticulture, they reduce fertilizer needs for cycads.
Applications: Studied for biofertilizer development; used in ornamental planting.
Velamen Roots
Velamen roots are aerial, adventitious roots with a spongy, multilayered velamen tissue, found in epiphytic orchids and some aroids. They absorb moisture from the air and anchor plants to hosts.
Morphology: Thick, white or silvery when dry, green when wet, with velamen covering a thin cortex. Root tips are active for absorption and attachment.
Functions:
- Absorption: Captures atmospheric water, dew, or rain.
- Anchorage: Secures epiphytes to bark or rocks.
- Protection: Velamen shields roots from desiccation and UV radiation.
Adaptations: - Velamen acts as a sponge, rapidly absorbing moisture during brief wet periods.
- Symbiotic fungi within roots enhance nutrient uptake in low-nutrient environments.
- Green chloroplasts in some roots support photosynthesis.
Examples: - Phalaenopsis (Phalaenopsis spp.): Velamen roots thrive in humid canopies.
- Vanda (Vanda coerulea): Roots absorb moisture in open air.
- Anthurium (Anthurium andraeanum): Roots anchor to rainforest trees.
Ecological Significance: Velamen roots enable epiphytes to dominate tropical canopies, increasing biodiversity and nutrient cycling. They trap organic debris, forming microhabitats. In horticulture, they require specific humidity for cultivation.
Applications: Critical in orchid propagation; studied for water-retention technologies.
Tuberous Roots
Tuberous roots are swollen, adventitious roots that store nutrients, distinct from tubers (stem-based). They form clusters from the stem base, supporting regrowth or propagation.
Morphology: Thick, elongated, or rounded, with starchy or fibrous tissues. Often fibrous externally, they produce buds for new shoots.
Functions:
- Storage: Stores carbohydrates or water for seasonal survival.
- Propagation: Buds on roots generate new plants clonally.
- Anchorage: Provides moderate stability in loose soils.
Adaptations: - High starch content supports rapid regrowth after dormancy.
- Bud formation enables vegetative reproduction in perennials.
- Protective skin reduces water loss and pathogen entry.
Examples: - Dahlia (Dahlia spp.): Tuberous roots store energy for flowering.
- Daylily (Hemerocallis spp.): Roots support clonal spread.
- Asparagus (Asparagus officinalis): Roots store nutrients for spears.
Ecological Significance: Tuberous roots ensure perennial survival in seasonal climates, supporting grassland and garden ecosystems. They provide food for herbivores and humans, with agricultural importance in ornamental and edible crops.
Applications: Used in horticulture for propagation; studied for starch storage mechanisms.
Root System Variations by Plant Life Form
Root systems also vary by plant life form (e.g., trees, shrubs, herbs, aquatic plants), reflecting adaptations to growth habits and lifecycles.
Tree Root Systems
Trees develop extensive taproot or fibrous systems, often transitioning from juvenile taproots to fibrous or buttress roots in maturity. They support massive above-ground biomass and long lifespans.
Morphology: Deep taproots in oaks or pines; fibrous networks in maples; buttresses in tropical species. Lateral roots may extend 2–3 times the canopy radius.
Functions:
- Anchorage: Supports tall trunks against wind and gravity.
- Absorption: Accesses deep or surface water and nutrients.
- Storage: Stores reserves for seasonal growth or stress recovery.
Adaptations: - Secondary thickening creates woody roots for durability.
- Mycorrhizal associations enhance nutrient uptake in forests.
- Buttress or prop roots stabilize in shallow soils.
Examples: - Pine (Pinus sylvestris): Taproot in youth, fibrous in maturity.
- Mangrove (Avicennia marina): Pneumatophores and prop roots.
- Baobab (Adansonia digitata): Thick taproot stores water in arid zones.
Ecological Significance: Tree roots prevent erosion, sequester carbon, and create forest soil structure. They support symbiotic fungi and fauna, driving ecosystem stability. In forestry, they influence timber quality and site selection.
Applications: Critical in agroforestry, reforestation, and urban planning.
Herbaceous Root Systems
Herbaceous plants (annuals, biennials, perennials) typically have taproot or fibrous systems, suited for short lifecycles or seasonal regrowth. They focus on rapid nutrient uptake and reproduction.
Morphology: Taproots in biennials (e.g., carrots); fibrous in annuals (e.g., grasses); tuberous or contractile in perennials (e.g., lilies). Shallow, often within 50 cm of surface.
Functions:
- Absorption: Maximizes nutrient uptake in fertile soils.
- Anchorage: Stabilizes smaller plants in loose substrates.
- Storage/Propagation: Supports regrowth or cloning in perennials.
Adaptations: - Rapid root growth suits short growing seasons.
- Shallow spread exploits surface moisture in rain-fed systems.
- Storage structures ensure survival in seasonal climates.
Examples: - Sunflower (Helianthus annuus): Fibrous roots for annual growth.
- Parsnip (Pastinaca sativa): Taproot stores energy for biennial cycle.
- Iris (Iris germanica): Rhizomatous roots propagate clonally.
Ecological Significance: Herbaceous roots enrich soils with organic matter, supporting microbial activity in grasslands and croplands. They stabilize soils in disturbed areas, aiding succession. In agriculture, they drive crop productivity.
Applications: Used in cover cropping, erosion control, and ornamental gardening.
Aquatic Root Systems
Aquatic plants develop adventitious or fibrous roots adapted to submerged, floating, or emergent lifestyles in wetlands, rivers, or lakes. They prioritize gas exchange and anchorage in water.
Morphology: Thin, fibrous roots in floating plants (e.g., water hyacinth); adventitious roots in emergent plants (e.g., cattails); reduced roots in fully submerged species. Often lack root hairs.
Functions:
- Anchorage: Secures plants in currents or muddy substrates.
- Absorption: Takes up nutrients from water or sediment.
- Gas Exchange: Aerenchyma tissues transport oxygen to submerged roots.
Adaptations: - Aerenchyma enhances buoyancy and oxygen supply in anaerobic conditions.
- Reduced root systems in submerged plants rely on foliar absorption.
- Dense root mats stabilize aquatic soils and filter water.
Examples: - Water Lily (Nymphaea spp.): Adventitious roots anchor in sediment.
- Cattail (Typha latifolia): Fibrous roots in marshes.
- Eelgrass (Zostera marina): Roots stabilize seagrass beds.
Ecological Significance: Aquatic roots support wetland ecosystems, filtering pollutants, stabilizing sediments, and providing fish habitats. They sequester carbon and mitigate flooding. In restoration, they rehabilitate degraded waterways. - Applications: Used in phytoremediation, aquaculture, and wetland conservation.
Adaptations to Environmental Conditions
- Arid Environments: Deep taproots (e.g., mesquite) or succulent storage roots (e.g., agave) access groundwater or store water, enabling survival in deserts.
- Wetlands: Pneumatophores, aerenchyma, or adventitious roots (e.g., mangroves) cope with flooding and low oxygen, supporting coastal and swamp ecosystems.
- Nutrient-Poor Soils: Mycorrhizal and nodulated roots enhance nutrient uptake, critical in forests, heathlands, or agricultural fields.
- Compacted Soils: Strong taproots (e.g., dandelions) penetrate hard layers, while fibrous roots exploit surface cracks.
- Saline Soils: Salt-excluding roots (e.g., mangroves) filter sodium, allowing growth in coastal or alkaline environments.
Root Systems in Specific Ecosystems
Root systems are tailored to ecosystem-specific challenges, influencing plant distribution and community dynamics.
Deserts
- Deep Taproots: Mesquite (Prosopis spp.) and acacias reach groundwater (10–20 m deep), surviving prolonged droughts.
- Succulent Roots: Agave and cacti store water in fleshy roots, supporting sparse rainfall.
- Shallow Fibrous Roots: Some grasses exploit brief rains, rapidly absorbing surface water.
Grasslands
- Fibrous Roots: Grasses (e.g., buffalo grass) form dense mats, binding soil and resisting grazing or fire.
- Taproots: Prairie forbs (e.g., coneflowers) access deeper moisture, coexisting with grasses.
- Nodulated Roots: Legumes (e.g., clover) fix nitrogen, enhancing grassland fertility.
Forests
- Taproots: Juvenile trees (e.g., pines) establish deep roots, transitioning to fibrous or buttress systems in maturity.
- Buttress Roots: Tropical trees (e.g., kapok) stabilize in shallow, nutrient-poor soils.
- Mycorrhizal Roots: Most forest trees rely on fungi for nutrient uptake, forming vast underground networks.
4. Wetlands
- Pneumatophores: Mangroves and cypresses aerate roots in anaerobic soils, supporting tidal or swamp ecosystems.
- Adventitious Roots: Cattails and water lilies anchor in sediments, absorbing waterborne nutrients.
- Aerenchyma: Aquatic roots transport oxygen, enabling growth in flooded conditions.
Tundra
- Shallow Fibrous Roots: Arctic grasses and sedges exploit thawed surface soils, avoiding permafrost.
- Taproots: Some forbs (e.g., saxifrage) penetrate shallow soils for stability and nutrient uptake.
- Mycorrhizal Roots: Enhance nutrient capture in cold, nutrient-poor soils.